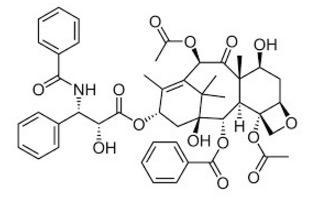
As if there weren't enough confusion in the universe of food vernacular (natural, GM-free, Earth-friendly...) there is a real word with real meaning that most people haven't even heard of—semisynthetic. And it has saved thousands of lives.
There can be no better example of what semisynthesis can accomplish than Taxol, a cancer drug that is the first line treatment for ovarian cancer, and is also approved for about a dozen other cancers, usually the ones that are most difficult to treat. The drug is on the World Health Organization list of essential medicines. It's that important.
The story of how Taxol became a drug is fascinating, and also illustrates the power of synthetic organic chemistry, without which the drug would not be available. Taxol was first isolated from the Pacific yew tree in 1962 by researchers from the National Cancer Institute (NCI). They were not looking for the Pacific yew, or any other tree in particular. Instead, the group was using one of the tools of drug discovery called natural products chemistry—a long, arduous process that involves collecting tens of thousands of specimens from living sources, grinding them up to make a crude extract, and then running a battery assays (tests), looking for any kind of biological activity that might be useful against the multiple targets (cancer, bacteria...) that are tested. Many drugs have been discovered this way (1).
But it is far from easy. Plants contain hundreds of chemicals, many found in minute quantities. So, the samples that are collected and tested are very complex mixtures. This is where the "fun" begins. If a crude extract shows anticancer properties, then chemists work to isolate and identify which particular chemical or chemicals are responsible for the activity of the extract. It is difficult to describe how challenging this is. Just separating the chemicals to isolate the right one can be a nightmare. But, the nightmare is just beginning. Next, the chemical structure must be elucidated (solved), which is done by use of a powerful arsenal of analytical instruments. This process can take years because natural products often have very complicated structures. Taxol is one of these.
The chemical structure of Taxol— An organic chemist's nightmare. Image: Wikipedia
To people who are not familiar with organic chemistry, the structure above may not mean much, but for an organic chemist it means only one thing: Rolaids. Although Taxol is not the most complex natural product out there, it's bad enough, which means that synthesizing it will be very difficult. During the time when the chemical that would later become Taxol was first isolated, analytical chemistry didn't have even a small fraction of the power it does today. This is reflected in the time required just to isolate the pure chemical and solve its structure— four years, (1967-1971).The amount of time required to get from the original isolation to that point was 19 years.
And then things got difficult.
As the drug was being evaluated in Phase I and Phase II clinical trials, two things became clear: 1) Taxol was providing previously unheard of response rates in ovarian cancer patients. 2) Supply was going to be an insurmountable problem if it was dependent upon the Pacific yew. Here's why.
Although Taxol was found in different parts of the Pacific yew, the largest amount was in the bark, and there sure wasn't much of it, even there. There was so little that the yield from crude extract to pure drug was 0.025 percent. To put this in prospective, let's use a cake. It takes 1.5 cups of flour to make one cake. But if the yield were 0.025 percent, it would take 6000 cups of flour to bake the same cake.
Worse still, stripping the bark kills the tree. At one point the NCI estimated that it would take the bark from 360,000 yew trees, all of which would then die, to treat all the ovarian cancer patients in the US—an impossibility. Another way of looking at this: one 40 foot, 200-year old tree yields only one-half of a gram of the drug. A typical dose is about one-tenth of a gram. Then the organic chemists got to work.
There are naturally derived drugs that face no supply problem because the living organism from which they are isolated are common and provide plenty of raw material. When this is not the case, the same drug can sometimes be made by a process called total synthesis. This is the specialty of organic chemists. They can start with very simple, commercially available chemicals and then, in a sequence of known (or sometimes invented) chemical reactions, sequentially build the simple materials into more and more complex structures, and eventually the drug, which will be identical to the purified material obtained from the plant.
Not so easy here. Because Taxol is so chemically complex, it was 1994 when the first total synthesis of the drug was published. In all, the process involved 40 sequential synthetic steps (2). As if this weren't challenging enough, no organic reaction is perfect. You never get 100 percent of the expected amount of the desired reaction product because byproducts (impurities) are formed in almost all chemical reactions. These may need to be removed before the next reaction in the sequence can be run. It is not uncommon to have to purify the product of every reaction that is run during a synthesis. So, no matter how well the chemistry works, some material is always lost in every step. The more steps, the more material gets lost. This can make a five-step synthesis a royal pain, but 40 steps??
A very clean reaction will provide about 90 percent of the expected product (this is referred to as a 90 percent yield), with the rest being impurities. A 90 percent yield is excellent, but not so common. Some reactions (we chemists HATE these) may have yields of only 10-20 percent, but you're stuck with them because there is no better method available for the particular transformation that needs to be done. The technical term for this is a #%%^% mess, and it can be very difficult to "fish out" the desired product from the all the (technical term) schmootz. And if you happen to be in the middle of a 40-step synthesis, even one reaction with a 20 percent yield means that you have to start with five-times the amount of material at the beginning of the synthesis. Technical term: That really sucks.
When there is important molecule to be synthesized, it is a sure bet that multiple research groups will be competing like crazy to be the first to finish the synthesis. Crossing the line is considered to be very prestigious. Sort of like the Chemistry Belmont Stakes. Indeed, there were dozens of chemistry groups in labs around the world that were in a very fierce competition to finish first (3).
Now there are about 10 different total syntheses of Taxol, each with its own design and strategy. What effect did these massive efforts have on drug supply? Zero.
This is because a 40-step linear synthesis (3) is not a practical way to make anything. Even if the entire synthesis consists of reactions with yields of 90 percent or better (and good luck finding this) the overall yield of final product will be 0.9 X 1040—0.014 percent. If the average yield is 80 percent—far more realistic— the overall yield will be 0.00013 percent. There is no way that any of these syntheses would ever provide enough Taxol.
Another method was clearly required, and it would combine both nature and chemistry. This is the famous semisynthesis of Taxol—arguably one of the most significant accomplishments in synthetic organic chemistry history.
(If you really hate your life and want to make it even worse, these notes of a group meeting from a chemistry class at Princeton compare and evaluate the synthetic pathways used by different groups to make the drug. Don't.
Enter Robert Holton. Holton, a professor of chemistry at Florida State University, didn't come up with the first total synthesis of Taxol—just the right one (4). And he didn't have to kill any Pacific yews. Instead, Holton took a chemical called 10-deacetylbaccatin (5), which is produced in larger quantities in the needles of the common English yew shrub (6). In what is perhaps the most lucrative four synthetic sequence ever, Holton patented the process and later sold it to Bristol-Myers Squibb, earning $351 million for Florida State and Holton, which touched off quite a battle between Holton and the school.
But, in the end, we got a very important drug, and one that is now easy to make. To paraphrase DuPont, "Better living though chemistry." Take that, NRDC.
The Holton semisynthesis of Taxol from 10-Deacetylbaccatin. The differences in the two molecules are depicted in red circles.
Notes:
(1) Examples include the cancer drug vincristine, codeine, digoxin, quinine.... many more.
(2) Many synthetic pathways can be designed so that different pieces of the molecule are made separately, and then joined together later on. This is called convergent synthesis, and is almost always better than sequential.
(3) If you happen to be a grad student or post-doc in one of these labs, god help you.
(4) Holton would later complete his own total synthesis of Taxol, but the four-step reaction sequence is far more important.
(5) Holton let nature do most of the work. 10-Deacetylbaccatin is a biological precursor to Taxol.
(6) Although the yew needles worked very well, researchers continue to look for better ways to obtain 10-deacetylbaccatin, for example, using cultured cells.